This is a cross-post of a couple of comments at the Physics Forums.
Meta Update (September 26, 2019): I kind of prefer the font below to the one I've been using for a while. Comments pro or con on whether I should change the default font in blogger if I can figure out how to do it are welcome.
Cross-Sections Of Interaction With What (And Self-Interacting Dark Matter Models)?
There are dozens of Dark Matter (DM) particle candidates.
And, of course, the answer also depends upon cross-sections of interaction with what (e.g. many self-interacting dark matter candidates have a cross-section of interaction of DM particles with other DM particles comparable to that of ordinary protons and neutrons with each other, but a tiny or non-existent cross-section of interaction with ordinary matter). The estimates of self-interaction cross-sections of DM with DM are based primarily upon observations of colliding galactic clusters such as the Bullet Cluster.
Experimental Exclusions For Dark Matter-Nucleon Cross-Sections
An easier and related question is what cross sections of interaction with ordinary matter nucleons are ruled out experimentally.
There are charts of cross-sections of interactions with nucleons (i.e. protons and neutrons) that have been excluded by a combination of multiple experiments. Where lines appear in the chart, cross-sections above those lines are ruled out by the experiment that the line is named after. The chart below is from a
July 31, 2015 paper by the CMS collaboration. Greater nucleon cross-sections have been excluded in the meantime, but this was the chart that I could pull up most conveniently.
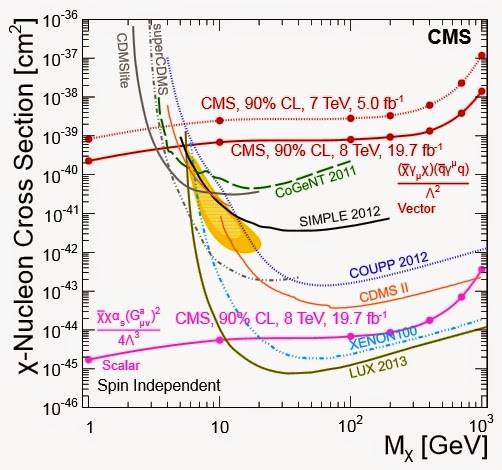
This second chart from May of 2018 (
via the Resonaances blog compiled from the sources linked therein) is more recent but excludes limits from the LHC such as those shown in the chart above (which are the strongest limits for lower mass dark matter particle candidates). The dotted blue line labeled "Z portal Cx=1" in the chart below is basically equivalent to the cross-section of interaction with nucleons of ordinary Standard Model neutrinos.
A
femtobarn (fb) is 10^-39 cm^2. An attobarn (ab) is 10^-42 cm^2. A zeptobarn (zb) is 10^-45 cm^2. A yoctobarn (yb) is 10^-48 cm^2.
The Combined Dark Matter-Nucleon Cross-Section Exclusions
Combining the charts, for Dark Matter Masses of 1-10 GeV as of 2015 LHC data, the exclusion for spin-independent interactions with spin=1 dark matter particles is roughly equal to the Z portal Cx=1 line and the exclusion for spin-independent interactions with spin=0 dark matter particles is roughly 1 zb at 1 GeV of dark matter particle mass and a slightly larger cross-section (10^-44 cm^2) at 10 GeV, gradually declining between the two points.
The reason that direct detection experiments like Xenon 1T are less powerful at excluding dark matter candidates at low masses is because distinguishing signals from neutrino flux backgrounds that are not precisely known becomes a challenge at those masses.
In general, cross sections of interaction of dark matter with nucleons is less than 1/1000th of the cross-section of interaction with neutrinos at most masses down to 8 GeV for almost all kinds of dark matter particles and down to 1 GeV for scalar (spin=0) dark matter particles that have spin independent interactions between dark matter particles and nucleons, but less restrictive for dark matter particles of under 8 GeV that do not have not spin-independent interactions or do not have spin=0.
Millicharged Dark Matter
Dark matter candidates with cross-sections of interaction with nucleons on the order of 1/1000th of the cross-section of interaction of neutrinos with nucleons are called "millicharged" dark matter particle candidates.
These are attractive candidates mostly because direct dark matter detection experiments do not rule them out.
There Is Not Credible Evidence Of Direct Dark Matter Detection
There is, in general, zero experimental evidence ever by any means that is confirmed by more that one experiment of any signal of dark matter particles, and there is no discovery level (five standard deviations from a null hypothesis) of a signal of dark matter particles. There were some isolated and inconsistent signals of dark matter-nucleon interactions at low statistical significance in some early direct dark matter detection experiments, but all of the signals have been conclusively ruled out by multiple other experiments of the same type with higher capacity to see such signals.
All of this is complicated by a need to explain the close correlation between inferred dark matter distributions and the distribution of ordinary (a.k.a. baryonic) matter in a galaxy or galactic cluster, which is strongly suggestive of some cross-section of interaction between dark matter particles and ordinary matter that is materially stronger than the neutrino-nucleon cross-section of interaction combined with the purely gravitational interaction of dark matter with ordinary matter assuming standard weak field general relativity. This is one reason among several that the current trend in dark matter particle proposals is to focus on "light" (i.e. less than 1 GeV) mass dark matter particle candidates such as "warm dark matter", "sterile neutrinos", and "axion-like particles."
WIMP Dark Matter
The earliest main candidate for dark matter was the WIMP (weakly interacting massive particles, mostly often the lightest supersymmetric particle), which was believed to have a particle mass between 10 GeV and 1000 GeV or so. In the strict sense of the term, a WIMP was weakly interacting in the technical sense of interacting only via the weak nuclear force (basically via Z boson interactions) with a cross-section of interaction equal to the neutrino with nucleons (since this was a property of electromagnetically neutral supersymmetric particles), and this narrow sense WIMP has been pretty much ruled out. But, in the broader sense, the "weakly interacting" aspect of a WIMP is defined in a more colloquial sense as very weak but non-zero, in which case it is ill defined and could have a smaller cross-section of interaction with a nucleon than a neutrino, in which case it is not ruled out by direct detection experiments.
Supersymmetric WIMPs are pretty much ruled out by direct dark matter detection experiments. There are really no widely considered theories devised for any reason, but to specifically fit direct dark matter detection experiments, that predict that millicharged WIMPs should exist.
Sterile Neutrino Dark Mattter
Sterile neutrinos have no tree level interactions with other matter at all, but have a tiny interaction indirectly because a true "sterile neutrino" oscillates into an ordinary active neutrino at some low probability and approximately speaking, that low probability times the cross-section of interaction of an active neutrino with a nucleon is an effective cross-section of interaction with nucleons for a sterile neutrinos. (I distinguish a "true sterile neutrino", because sometimes the term "sterile neutrino" is used less discriminatingly as a dark matter particle with no cross section of interaction via the strong, electromagnetic or weak forces even if it does not oscillate with active neutrinos in some way.)
True sterile neutrino dark matter is favored most strongly with masses on the order of 1 eV (based upon the "reactor anomaly" which is a subject for another post), and almost always significantly less than 1 keV. So, experiments are not sufficiently sensitive to detect the predicted cross-section of interaction that a sterile neutrino should have with ordinary matter.
Thermal Freeze Out Dark Matter And Implications For Cross-Sections Of Interaction
Most (but certainly not all) dark matter particle candidates are presumed to have "frozen out" after the Big Bang at a characteristic temperature that relates dark matter particle mass and dark matter particle mean velocity. In those models "colder" dark matter particles (i.e. dark matter particles with lower mean velocity) are more massive, while "warmer" dark matter particles (i.e. dark matter particles with higher mean velocity) are less massive.
Normally "cold dark matter" in the strict sense, when referring to thermal freeze out dark matter particles, refers to particles 1 GeV or more, "warm dark matter" involved particles in the 1 keV mass order of magnitude, and "hot dark matter" involves particles with relativistic mean velocities that typically have masses comparable to neutrinos. But, the mass-mean velocity relationship breaks down for dark matter particles that are not generated by thermal freeze out.
I bring up this distinction because galaxy dynamics and the amount of structure in the universe largely rule out both colder dark matter particles, and hot dark matter particles, as serious dark matter candidates. Instead, thermal freeze out models taken together with galaxy dynamics and large scale structure tend to favor "warm dark matter" with masses on the order of a keV, and that small mass does not have a terribly tightly constrained cross-section of interaction with ordinary matter since it is so hard to distinguish between the neutrino background and the dark matter signal.
Non-Thermal Freeze Out Models
Two kinds of dark matter particles are that are good examples of models that are not thermal freeze out models. These are sterile neutrino models and axion-like particle models.
Primordial Black Hole Dark Matter
Primordial black hole dark matter candidates (i.e. black holes with an initial mass of less than the roughly three stellar masses at which they form naturally in non-Big Bang conditions), which are ruled out except in a narrow mass range as the primary component of dark matter (roughly big asteroid masses), of course, have extremely high (basically 100%) cross-sections of interaction with nucleons, but there are far fewer of them out there since they would be much more massive than other dark matter particle candidates.
If dark matter is too "hot" (i.e. relativistic speeds and typically neutrino scale masses), you get too little structure (e.g. no galaxy formation). But, if dark matter is too "cold", you get too much structure relative to what is observed (e.g. far more tiny galaxies than we observe, and far more substructures within normal sized galaxies than we observe). Dark matter particles have to be "warmish" to produce the right amount of structure.
W Boson and Z Boson Decay Constraints
Because of the way that the weak force operates, any particle that interacts via the weak force and has a rest mass of less than 45 GeV, give or take, must be produced in the decay of W and Z bosons that carry the weak force. Basically, in quantum physics everything that is possible must happen with some fixed probability. This is why we know, for example, that there can be only three kinds of "active" neutrinos (i.e. neutrinos that interact via the weak force) with masses of 45 GeV or less.
This is a huge gap considering that the heaviest active neutrino is directly constrained by a recent experiment to have a mass of only
a little more than 1 eV (with cosmology and neutrino oscillation data suggesting masses even lower of under 0.1 eV) mostly due to experimental imprecision with the best fit value that is negative, which is more than 30 billion times smaller than the upper limit of active neutrino masses that are ruled out by W and Z boson decays. In five years of continued data gathering, the direct absolute neutrino mass threshold experimentally should be as low as 0.35 eV if neutrinos are indeed less massive than that as cosmology and neutrino oscillation data suggest (incidentally, the same experiments measuring absolute neutrino mass directly are also used as warm dark matter direct detection experiments but have found nothing so far on that front).
But, Standard Model particles fully account for all such decays to a precision that can rule out the existence of any particles of 45 GeV or less than interact via the weak force with the strength of the other massive fundamental particles in the Standard Model (i.e. all fundamental fermions other than the top quark). So, this provides an independent basis to rule out low mass dark matter particles that interact via the weak force that are not millicharged dark matter, and are also not dark matter that doesn't interact via the weak force at all.
Higgs Boson Decay Constraints
Similarly, if there were a fundamental particle that is not part of the Standard Model with a mass of between 45 GeV and 62.5 GeV that derived its mass through the Higgs mechanism, this particle, rather than the bottom quark, would be the dominant means by which the Higgs boson decays and couldn't be missed at the LHC so far. Indeed, this would be true of any fundamental particle that derived its mass from the Higgs mechanism with a mass from 4.3 GeV (just above the bottom quark mass) to 62.5 GeV (about half the Higgs boson mass). So, observed Higgs boson decays are inconsistent with a dark matter particle that derives its mass from the Higgs mechanism for a large swath of the cold dark matter mass range. So, if there is a dark matter particle with a mass in this mass range, it would instead have to acquire its rest mass via some other mechanism.
Taken together, the W boson, Z boson and Higgs boson constraints combined are among the strongest constraints on the properties of dark matter particles with masses of less than about 8 GeV.
Note On Inconsistent Use Of The Term "Cold Dark Matter"
Note also that there is a terminology disconnect. People who talk about potential dark matter particle candidates distinguish between cold dark matter, warm dark matter and hot dark matter. But, the "cold dark matter" in the lambdaCDM "standard model of cosmology" has a definition loose enough to encompass both warm dark matter and cold dark matter, so long as the dark matter particles don't interact very much at all with either other dark matter particles, or with ordinary matter like nucleons.
No Dark Matter Particle Theory Works In All Circumstances, And There Are Other Alternatives, Although None Of Them Work In All Circumstances Either.
Also, to be clear, there are several ways to explain dark matter phenomena: dark matter particles alone, dark matter particles that have fifth force type interactions either with each other or ordinary matter or both, modifications of gravity alone, or a combination of one or more of the previous possibilities.
As this brief summary suggests, the parameter space available in which dark matter particles alone can provide an explanation is narrow and is on the verge of being over constrained, if it isn't already. If so, it is entirely possible that dark matter particles are not the explanation for dark matter phenomena, in general, or are only part of the explanation. Instead, we also need a new force or a modification of gravity, or both.
This is unfortunate, because while lambdaCDM does a good job of explaining cosmology scale phenomena with a small number of parameters and unambitious assumptions, it can't easily be extrapolated to galaxy cluster and galaxy scales because at those scales plain vanilla cold dark matter doesn't fit the data very well.
In fact, there are no widely supported theories in existence with either a dark matter particle component, or a modified gravity component, that adequately explain the dark matter phenomena data at all scales and in all circumstances.